Research
Overview
Despite breakthroughs in the scientific understanding of deformation processes, the mechanical properties of engineering materials remain limited to fractions of their theoretical values. One approach to unlocking improved material performance is through nanostructuring (i.e., controlling the organization of a material at the nanoscale). However, the preservation of nanostructured properties at macroscales remains a pervasive challenge. In the Advanced Materials and Microstructures Lab, we are interested in answering this overarching research question: how can materials be engineered at the nanoscale to translate into macroscale structures with unprecedented properties?. The breadth of this question touches on a diverse set of material systems including: high entropy materials, nanostructured metals, 2D materials, and bioinspired materials.
Deformation mechanisms in engineering alloys
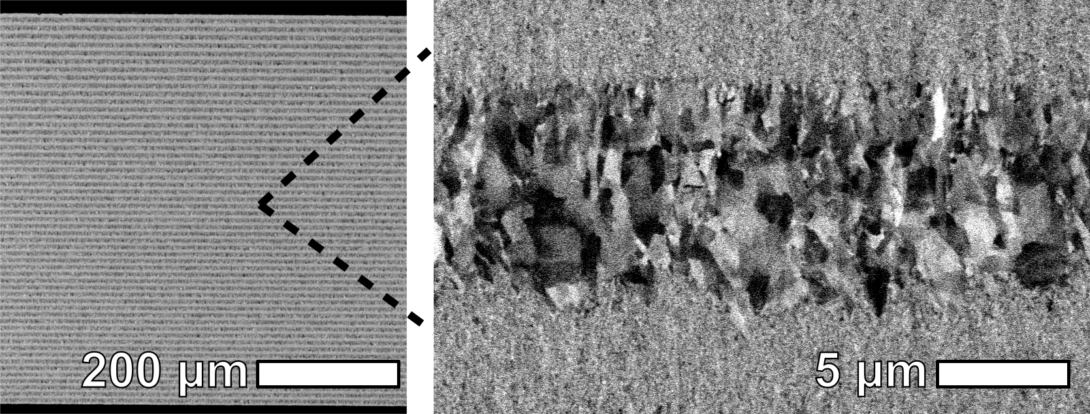
Deformation mechanisms describe the physical processes by which materials accommodate plasticity. The aggregated effect of these mesoscale processes emerges as the mechanical properties that are measured at the experimental scales. Consequently, materials can realize orders of magnitude increases to their mechanical properties through careful engineering of deformation behavior. Yet, engineering the preferred activation of specific deformation mechanisms is non-trivial and is ultimately determined by a complex interplay between intrinsic and extrinsic material properties. At the AMML, we are investigating new approaches to manipulating the competition between deformation mechanisms through complementary experimental-computational techniques. Our work in this area involves a wide cross-section of systems including high entropy alloys, heterogeneous multilayers, and nanostructured materials. For examples of previous works by members of the lab on this topic, please see our publications.
Key References:
- R. Jagatramka, C. Wang, and M. Daly, Journal of Applied Physics, 133 055107, 2023.
- R. Jagatramka, C. Wang, and M. Daly, Computational Materials Science, 214 111763, 2022
- J. Ahmed and M. Daly, Materials Science and Engineering: A, 820 141586, 2021
- M. Daly, S. Haldar, V. K. Rajendran, J. McCrea, G. D. Hibbard, and C. V. Singh, Materials Science and Engineering: A, 771 138581, 2020.
2D Materials
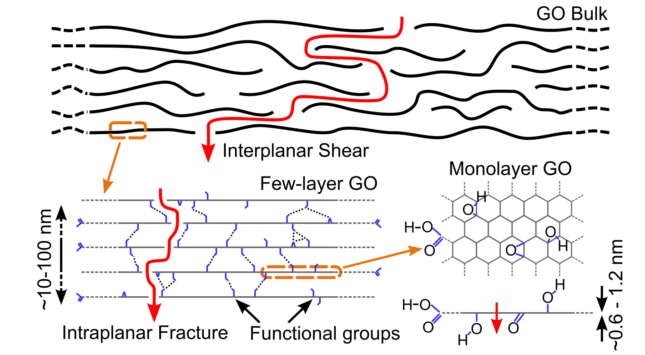
2D materials (i.e., materials that are one plane of atoms thick) are known to possess unprecedented physical properties. New structural motifs may therefore be envisioned, which leverage the extraordinary properties of 2D materials as building blocks in scalable lightweight structures. However, the realization of these materials systems inherently requires the interrogation of material properties and mechanics laws at the atomic scale, which presents a number of unique challenges for the materials science community. At the AMML we employ novel characterization protocols to reveal the length-scale dependent physical properties of these material systems. For examples of previous works by members of the lab on this topic, please see our publications page.
Key References:
*equal contribution
- G. Wang, H. Hou, Y. Yan, R. Jagatramka, A. Shirsalimian, Y. Wang, B. Li, M. Daly and C. Cao, International Journal of Extreme Manufacturing, 5 032002, 2023.
- M. Daly*, C. Cao*, H. Sun, Y. Sun, T. Filleter, and C. V. Singh, ACS Nano 10 1939-1947, 2016.
- C. Cao*, M. Daly*, B. Chen, J. Y. Howe, C. V. Singh, T. Filleter, and Y. Sun, Nano Letters 15 6528-6534, 2015.
- C. Cao*, M. Daly*, C. V. Singh, Y. Sun and T. Filleter, Carbon 81 497-504, 2015.
Bioinspired Materials
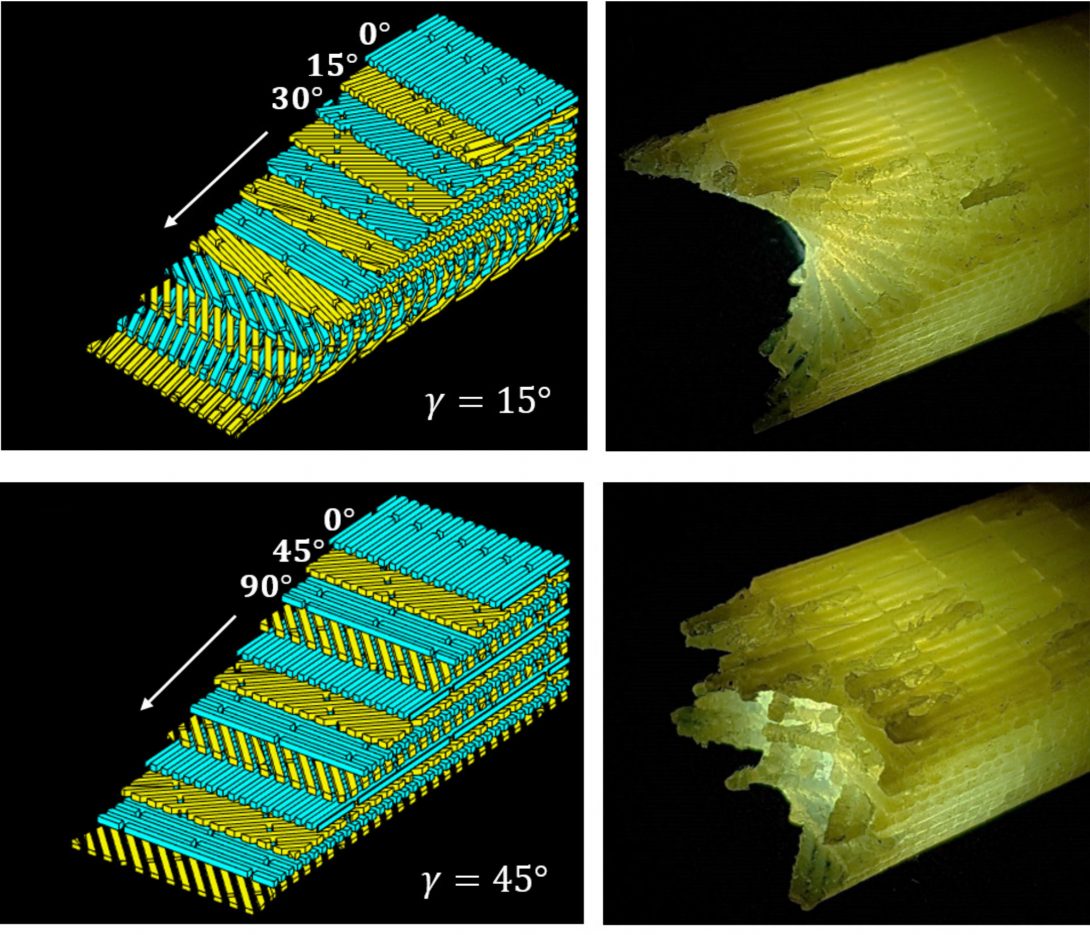
Through the process of evolution, nature has developed efficient microstructure design strategies that deliver high performance materials systems. One of the keys to the success of biological materials is the leveraging of nanostructured phases as constituents in structural materials. In this sense, nature has achieved an engineering feat that has eluded materials scientists. Namely, nature has developed voxel-level processes to fabricate complex microstructures that avoid the normal performance pitfalls encountered when scaling nanostructured materials. With the advent of additive manufacturing, voxel-level control of microstructure is now available to materials scientists – enabling synthetic replication of high performance biological systems. At the AMML, we are interested in utilizing additive manufacturing technologies to design microstructures with unprecedented properties. For examples of previous works by members of the lab on this topic, please see our publications page.
Key References:
- A. Zaheri, J. Fenner, B. Russell, D. Restrepo, M. Daly, D. Wang, C. Hayashi, M. A. Meyers, P. D. Zavatierri, and H. D. Espinosa,
Advanced Functional Materials, 1803073, 2018. - M. J. Chon, M. Daly, B. Wang, X. Xiao, A. Zaheri, M. A. Meyers, and H. D. Espinosa, Journal of the Mechanical Behavior of Biomedical Materials, 76 30-37, 2017.
Shape Memory Alloys
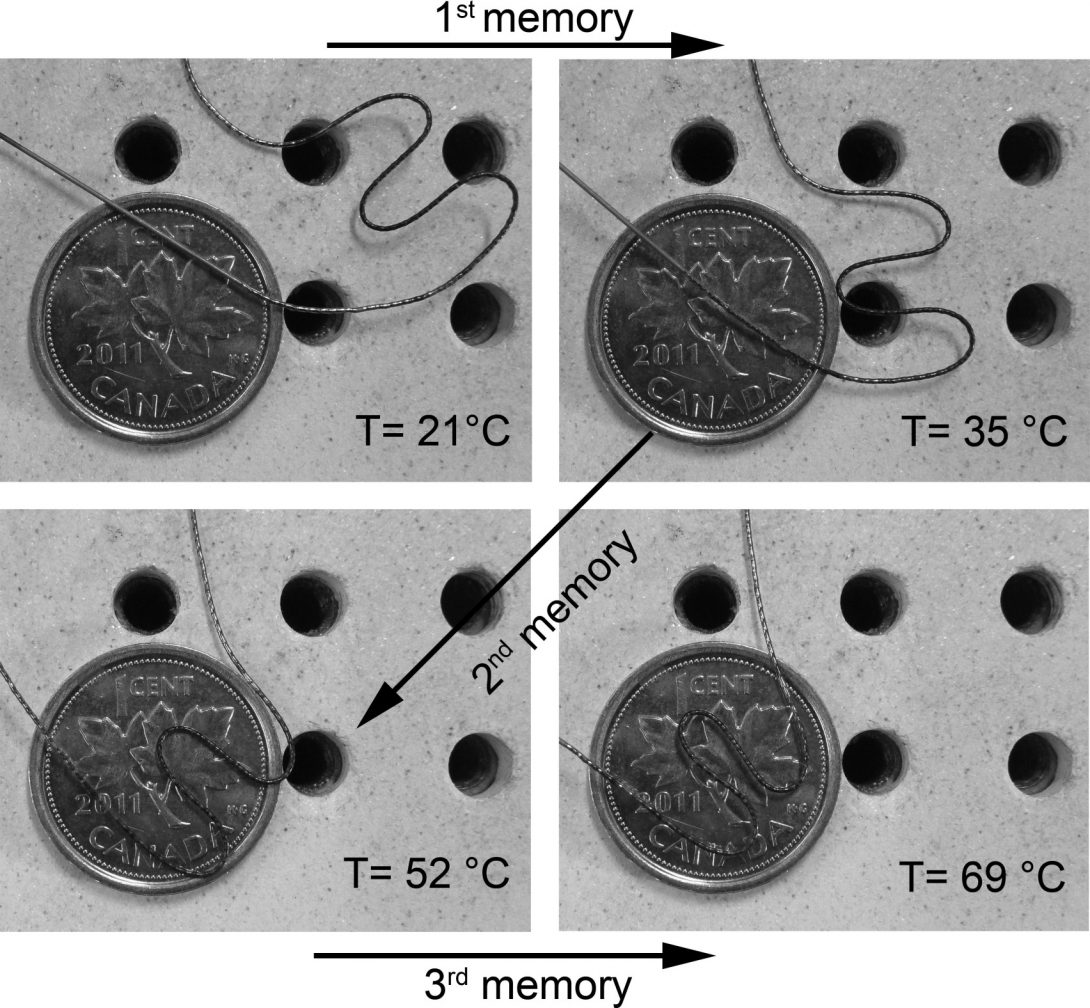
Shape memory alloys (SMA)s are a class of materials that can undergo shape changes when heated above a characteristic transformation temperature. Traditional SMAs are limited to one transformation temperature, meaning that they can only “remember” one specific shape. At the AMML we are investigating techniques to overcome these limitations, leading to multifunctional smart devices that can remember several shapes. This unprecedented functionality enables finds applications in smart actuation systems in the automotive, aerospace, and biomedical industries. For examples of previous works by members of the lab on this topic, please see our publications page.
Key References:
- M. Daly, A. Pequegnat, Y. N. Zhou, and M. I. Khan, Journal of Intelligent Material Systems and Structures, 28 984-990, 2013.
- M. Daly, A. Pequegnat, Y. Zhou, and M. I. Khan, Smart Materials and Structures, 21 045018, 2012.